The Future of Aviation: Aircraft Electrification
The following is a guest post by Jason D’souza, a recent MSc Graduate in Aerospace Vehicle Design from the University of Cranfield in the UK. Jason is a long-term reader of the blog and reached out to me about the idea of a guest post on electric aviation. As I have covered the prospects of electrification on my podcast, and believe that electrification will have profound effects on the aviation industry just like the jet engine did post-WWII, I thought this would be a great opportunity to get a young engineer with fresh ideas to write about this exciting area. And, of course, if you have any questions or want to discuss the topic matter, please feel free to reach out to Jason directly.
Introduction – The Need for Electric Propulsion
In the automotive industry, electrification is developing at a fast rate. In the UK, both electric and hybrid cars are becoming a common sight on roads and electric charging ports are ever-more common in car parks. Electric vehicles offer a better solution towards a cleaner, greener environment than their diesel/petrol counterparts, as well as reducing noise pollution for those living in busy residential areas. So, when will air travel and air freight follow this electric journey?
Certainly, aircraft emissions degrade air quality and have an increasing effect on global warming as most emissions occur at high altitudes. According to the European Union [1], aviation accounts for approximately three percent of the EU’s total greenhouse gas emissions, and more than two percent of global emissions. Although air travel currently accounts for only a small portion of global emissions, air travel is recognized as one of the fastest growing contributors, as elucidated in a recent study in the Atmospheric Chemistry and Physics journal. But by what mechanisms is aviation actually effecting the climate?
Aircraft engines do not only produce plenty of CO2, but also exhaust nitrous oxides, NOX, fine particulate matter, PM25, and ozone, O3. CO2 emissions are the most significant contributor to climate change and have the same effect regardless of altitude emission. However, at higher altitudes, NOX emissions are particularly effective in forming O3, thereby causing a greater global warming effect than would be the case at lower altitudes.
To put this into perspective, if you’ve ever played with a Carbon Footprint Calculator and plugged in a return trip from London to New York, this trip balloons your carbon footprint by more than 2000 pounds of CO2, nearly equivalent to the level of emissions incurred by heating an average European household for an entire year [2]. Reducing the CO2 impact of aircraft should be addressed as early as possible, as future regulations may impart even stricter regulations on environmental targets that will only be achieved by the use of the latest, and hence most expensive, technologies.
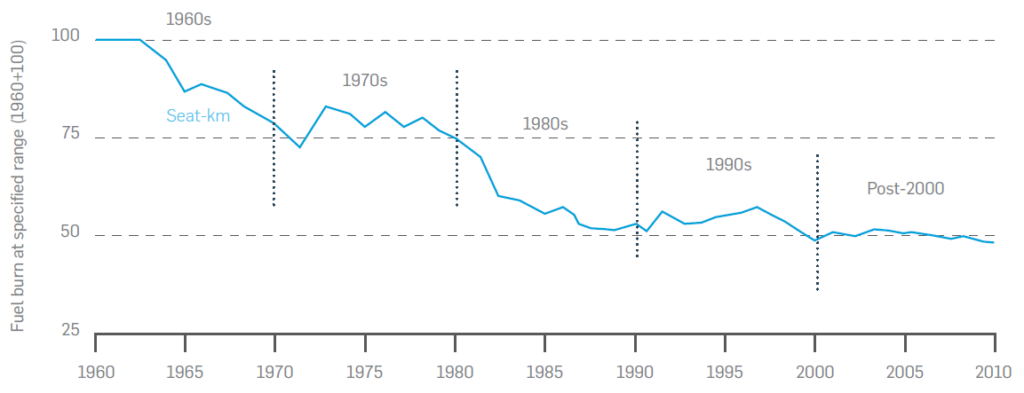
Hence, there is a clear incentive for cleaner, greener air mobility: the reduction of CO2, NOX, PM25 and O3 emissions, reduced noise and better fuel consumption. Currently, we have reached a plateau in terms of improving aircraft fuel efficiency and reducing emissions. By introducing electric battery-powered aircraft, an opportunity exists to reduce potentially harmful emissions even further and to make air travel quieter in urban areas. But is electric flight actually possible given the technological challenges?
Electric Innovations: Past, Present and Future
Many industry giants are researching and developing new prototypes and concepts for electrification. Commercially, the Airbus A350 and Boeing 787 increasingly use battery power for several of their on-board systems to form an approach described as the ‘More-Electric Aircraft’ (MEA). At the same time, both Airbus and Boeing are actively developing methods to progress towards full electric propulsion. The strategy is to begin with a hybrid option first, with energy still provided by hydrocarbon fuels, and then progress to an all-electric system with batteries to provide energy for propulsion.

In technical terms, the main challenge that hybrid or electric aircraft, such as the Airbus E-Fan X, Zunum Aero and Wright Electric, are faced with is power-to-weight ratio or specific power. To make an all-electric system a reality, a battery with greater energy density than currently available, with a longer service life and improved reliability is needed. However, historic trends and current understanding of aircraft development cycles suggest that battery technology will not reach the required technical level and production rates until the 2030s to support even current aircraft scales.
Potential Propulsion System Architectures
In the short term, we will probably witness a ‘More Electric-Hybrid’ commercially sized aircraft rather than an ‘All-Electric’ alternative. This ‘More Electric-Hybrid’ version could deliver real benefits through reduced emissions and allow for the development of motors and power electronics for the transition to fully electric operation. Let’s examine, on a system level, what a hybrid powered solution might look like.
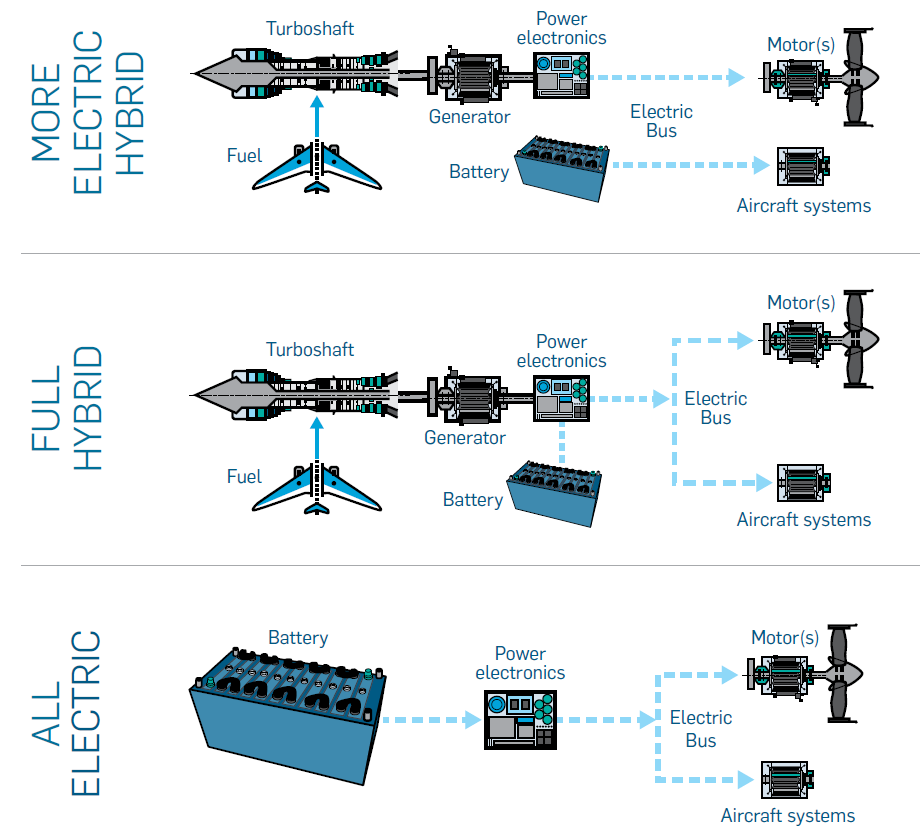
Using an existing gas turbine with traditional jet fuel (kerosene) mixed with biofuels to drive an electric generator creates a simple and well-understood solution. However, new power electronics systems are likely to be required to control and transfer the electrical energy to electric motors to provide the requisite thrust.
If only batteries are used to power the remaining systems on the aircraft, then the architecture is known as a ‘More Electric-Hybrid’ solution. However, the power available from the generator and batteries can also be combined to power all aircraft systems to form a ‘Fully-Hybrid’ solution with each energy supply augmenting the other in different ratios during various flight phases. But, if kerosene is still the source of energy, then where does the reduction in emissions come from?
The aircraft’s flight is affected by the varying thrust requirements needed for take-off, climb, cruise, and altitude changes. Current jet engines are designed to operate in all conditions but do not always function optimally and efficiently in all flight segments. In a hybrid solution, the gas turbine can be isolated from changes in operational conditions and continuously run at an optimized speed to power an electric generator, which then drives the power electronics that provide electrical energy.
The main danger in this scenario is relying on unproven power distribution systems and electric motors. Indeed, one of the stated objectives of the Airbus E-Fan X program is to address this uncertainty with regards to electric power systems. Further advancements can be made by switching the kerosene-powered gas turbine and generator to battery power, thereby forming an ‘All-Electric’ system. In this case, the power electronics used for the hybrid solution will need to be adapted, but the propulsive element could remain the same.
Electric Motor Development
To date, Siemens has developed a 50 kg electric motor SP260D that delivers 260 kW power output—five times more than comparable hybrid electric propulsion systems of equal weight. However, this is still a long way away from the power required for a commercial aircraft to fly at acceptable speeds (2-50 MW). Nonetheless, Siemens has established its electric propulsion systems business through the development of the eAircraft program, now acquired by Rolls Royce.
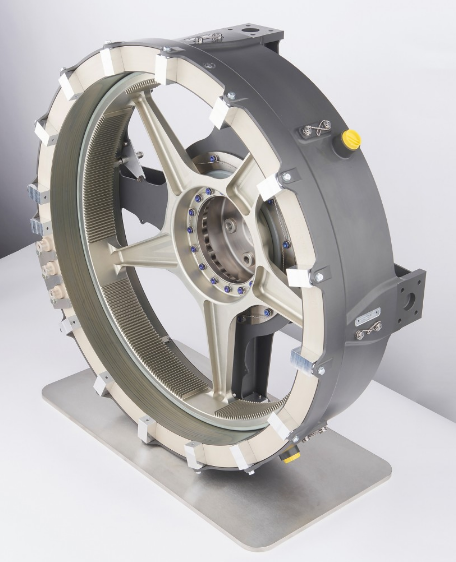
So, with improved technology, can enough power be generated? Currently, the answer to this question isn’t clear, keeping in mind changes to the overall aircraft weight, safety considerations for electrical systems, wiring routes, electrical interference, and thermal environment created by batteries.
A High Aspect-Ratio Wing Design
Aircraft wings are designed to withstand aerodynamic loads and carry jet fuel distributed across the span. For an electric aircraft, would there be any benefit of carrying batteries within the wings instead?
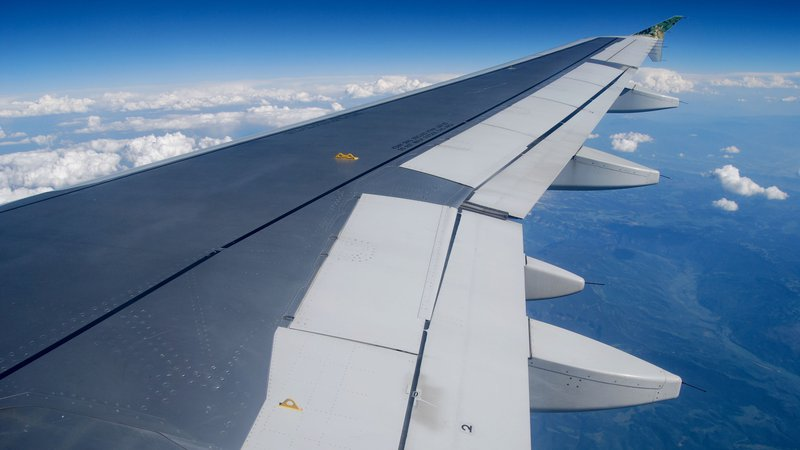
One positive side-effect of electrification would be that the wing’s mass remains constant during flight (unless using lithium air batteries), creating novel opportunities for aeroelastic tailoring and aerodynamic optimization by allowing wings to become longer and thinner (better lift-to-drag ratio). A current challenge of designing long and slender wings for conventionally fueled is the onset of ‘aeroelastic flutter’, an unstable interaction between airflow and the wing’s elasticity that causes a buildup of oscillations and potential wing failure. With strategically placed batteries, thinner and longer wings could emerge as a viable design that doesn’t succumb to flutter, thereby improving aerodynamics and paving the way for radically different aircraft configurations.
The Need to Reduce Aircraft Mass
What happens to the weight of the airframe in a move towards hybrid or full electrification? In a hybrid system, the full propulsion and electrical system, combined with all the technical operation equipment inside the aircraft, will probably increase the mass of the airframe. Furthermore, as the aircraft is no longer shedding mass via fuel burn during flight, the landing weight of aircraft is likely to be higher than it is today. To compensate for these effects, reductions in the airframe mass are probably required to facilitate a fully electric system. Indeed, designing lighter airframes is a huge challenge, putting great requirements on future research on materials science to determine the full capabilities of next-generation metallic alloys and fiber-reinforced plastics.
Funding Resources
The best solutions often come from coordinated efforts to ensure a collaborative, knowledge-sharing approach that leads to conformity in standards. The UK is a major player in Aerospace Research & Development and is involved in extensive research networks, partnerships, and bids to numerous funding bodies working on developing the technology for the future of air travel.
These funding bodies include the Engineering and Physical Sciences Research Council (EPSRC), as well as the Aerospace Technology Institute (ATI) established between industry and the government to develop the UK’s technology strategy through £3.9 billion of investment. Another influential body is the Air Transportation Systems Lab, which collaborates on research projects with the UK and overseas universities.
Lastly, the largest European research program developing innovative technologies aimed at aircraft emission and noise reductions, known as the Clean Sky Initiative, has a €4 billion budget and has backed several projects like Airbus’ BLADE and Safran’s Contra Rotating Open Rotor engine.
Regulation
There is a need for new regulations addressing emerging technologies, platforms and systems related to electrification. Firstly, as new technologies are created in the field of electric aviation, each technology will need regulatory backing to determine the airworthiness of the technology. This is critical to establish broad regulatory acceptance for enabling technologies like high-power batteries, voltage distribution systems and boundary layer ingestion.
Subsequently, regulation will be critical to enable new platforms. Regulation and certification procedures for radically new architectures such as distributed fans will be required to enable the full potential of Electrical Propulsion. As a first step, both the Federal Aviation Administration (FAA) and the European Aviation Safety Agency (EASA) are currently opening doors for electrical propulsion. For example, a key change in FAA Part 23 and EASA CS23 came into effect in 2017, allowing larger classes of general aviation aircraft to legally fly non-traditional engine types. This not only opens revenue potential for developers in general aviation, but this platform category is a key stepping stone to even larger architectures. There is, however, a long journey ahead and regulation must keep a-pace with the technological evolution of electric aircraft.
In April 2018, these new regulations provided the opportunity for Siemens to test the Magnus eFusion at Matkópuszta airfield in Hungary, using a Siemens SP55D electric motor and a FlyEco diesel engine to allow for silent take-off and landing with an extended range. The full electrical propulsion system is developed by the Siemens eAircraft program. Furthermore, a Los Angeles-based company Ampaire recently demonstrated its electric hybrid propulsion system at Camarillo Airport in California with its maiden flight test in June 2019. Their technology was adapted to the Cessna 337 Skymaster and is already considered by some as the largest hybrid-electric aircraft to have ever flown.
There’s Turbulence Ahead
Certainly, the biggest challenge is integration. For example, when designers and engineers have new technologies ready for flight, how does the industry support in-service operation? As an aviation company, how will business models need to adapt over the next twenty years? When is the best time to start thinking about how to integrate these new business models with the shift in aircraft architecture? Even though some of these changes are still years away, it pays to contemplate the disruptive nature of these changes now, and to consider strategies of how the industry can ready itself to face up to these coming challenges.
Additional Thoughts
Keeping in mind the potentially great opportunity aircraft electrification presents, Maintenance and Servicing Organizations (MRO’s) should be reconsidering their operations to address questions like what kind of physical infrastructure will be needed going forward? Topics for consideration range from how many spare batteries will need to be stored at airports and the most optimal location of recharging points, to how long it will take for the batteries to be charged or changed in between flights. The latter will be a considerable consideration for airlines, as an electric aircraft will not be earning money when it is recharging.
With regards to traffic control, what happens in the case of diversions and re-routing? Will regional electric aircraft take priority over long-range gas-powered aircraft? Or could quieter aircraft lead to additional airports in closer vicinity to cities, such that regional and long-range aircraft won’t share the same airspace? Finally, a major challenge will be instilling confidence in passengers about the safety of this new technology.
Conclusion – Where Do We Stand?
The continuing growth of the aviation sector has buoyed production rates and has led Airbus and Boeing to establish themselves as the key players in the aviation market. This duopoly has only been reinforced by recent mergers and acquisitions of smaller operations (Bombardier and Embraer). The shift to electrification poses a risk to incumbents, as this new paradigm in propulsion levels the playing field for new entrants entering the market.
At the same time, both incumbents and new players will face key technical barriers, such as improving the current energy storage capacity per unit weight of batteries, as well as light and efficient electrical generators, motors and power electronics able to convert, condition and switch to high voltage power.
On the regulatory side, airworthiness authorities will have to find approaches to certify novel aircraft architectures as for over fifty years commercial aircraft have not deviated from the gas turbine powered architecture. The Advisory Council for Aeronautics Research (ACARE) has brought about a united sense of purpose across the European aviation sector to shift towards greener solutions and has set challenging goals, such as achieving seventy-five percent CO2 emission reductions per passenger.
These challenges mean that we will probably witness a ‘More Electric-Hybrid’ commercially sized aircraft in the near future rather than an all-electric alternative. This could, however, still deliver real benefits through reduced emissions and allow for the development of motors and power electronics for the switch to fully electric operation in the far-reaching future.
Further Reading
Roland Berger GMBH (2017). Aircraft Electrical Propulsion – The Next Chapter of Aviation?.
References
[1] European Commission. Reducing emissions from aviation, accessed 19/08/2019.
[2] I Herbert and J Brown (2006). Your carbon footprint revealed: Climate change report finds we each produce 11 tons of carbon a year – and breaks down how we do it, accessed 19/08/2019.
[3] D Rutherford (2011). Overturning conventional wisdom on aircraft efficiency trends. The International Council on Clean Transportation, accessed 19/08/2019.
[4] J Domone (2018). The challenges and benefits of the electrification of aircraft. Atkins, accessed 19/08/2019.
[5] M Price (2018). Current and emerging trends in the aerospace sector. Atkins, accessed 19/08/2019.
[6] Siemens (2018). Electric Flight. Accessed 19/18/2019.
[7] C Choi (2017). The 100-Year-Old Idea That Could Change Flight. PBS Nova, accessed 19/08/2019.
Related Posts
One Response to The Future of Aviation: Aircraft Electrification
Leave a Reply Cancel reply
This site uses Akismet to reduce spam. Learn how your comment data is processed.
Sign-up to the monthly Aerospaced newsletter
Recent Posts
- Podcast Ep. #49 – 9T Labs is Producing High-Performance Composite Materials Through 3D Printing
- Podcast Ep. #48 – Engineering Complex Systems for Harsh Environments with First Mode
- Podcast Ep. #47 – Möbius Aero and MμZ Motion: a Winning Team for Electric Air Racing
- Podcast Ep. #46 – Tow-Steered Composite Materials with iCOMAT
- Podcast Ep. #45 – Industrialising Rocket Science with Rocket Factory Augsburg
Topics
- 3D Printing (4)
- Aerodynamics (29)
- Aerospace Engineering (11)
- Air-to-Air Refuelling (1)
- Aircraft (16)
- Autonomy (2)
- Bio-mimicry (9)
- Case Studies (15)
- Composite Materials (25)
- Composites (7)
- Computational Fluid Dynamics (2)
- Contra-Rotation (1)
- Design (2)
- Digitisation (2)
- Drones (1)
- Education (1)
- Electric Aviation (11)
- Engineering (23)
- General Aerospace (28)
- Gliders (1)
- Helicopters (3)
- History (26)
- Jet Engines (4)
- Machine Learning (4)
- Manufacturing (12)
- Military (2)
- Modelling (2)
- Nanomaterials (2)
- NASA (2)
- New Space (11)
- News (3)
- Nonlinear Structures (1)
- Novel Materials/Tailored Structures (14)
- Personal Aviation (5)
- Podcast (45)
- Propulsion (9)
- Renewable Energy (2)
- Renewables (1)
- Rocket Science (17)
- Satellites (8)
- Shape Adaptation (1)
- Smart Materials (1)
- Space (12)
- Space Junk (1)
- Sport Airplanes (2)
- Startup (19)
- STOL (1)
- Structural Efficiency (5)
- Structural Mechanics (1)
- Superalloys (1)
- Supersonic Flight (2)
- Technology (18)
- UAVs (2)
- Virtual Reality (2)
- VTOL (3)
- Privacy & Cookies: This site uses cookies. By continuing to use this website, you agree to their use.
To find out more, including how to control cookies, see here: Cookie Policy
Your article is slightly misleading by stating that:
“…Large reductions in fuel burn are seen from 1960 up to 1990s. Since then, further reductions have been modest, despite the development costs of new aircraft continuing to rise [3].”
The major improvements in aircraft propulsion efficiency you mention to be seen from 1960 to 1990 were actually driven only by the 1st (1973) and 2nd (1979) Oil Crisis. However later the engine developed with the highest efficiency (PW2000) was neglected by the market due to its high cost.
Presently the P&W Geared Turbofan technology that has proven a reduction of 16-17% in TSFC compared to previous technology CFM56 and V2500 engines (and a >20% reduction in Seat-Mile SFC) is again disfavoured by market forces that prefer a “cheaper solution”.
Please check the Fuel Consumption of the Airbus A220!